Fe (II) and Mn (II) oxidation
Found in quiet water, edge of lakes
Orange, red surface film or floc-like, slimy deposits in slow moving water
or pond. This color indicates the presence of oxidized iron.
Genera that exhibit the property: Chemollithoautotrophic iron oxidation
-Thiobacillus and Leptothrix (Beta Proteobacter) or by photoferrotrophy Rhodomicrobium
-alpha proteobacter, Chlorobium, Chloroflexus
Chemollithoautrophy:
Thiobacillus also involved in sulfide respriation (see box below)
Oxidation of ferrous iron occurs anaerobically with nitrate (pH 7)
Photoferrotrophy:
anoxygenic phototrophic bacteria
Authors for literature search: Konhauseretal, Hiesing et al, 1998, 1999
Leptothrix
is a well know genus that oxidizes FeII, (These are not photosynthetic. Many
of the other Fe oxidzers are photosynthetic.) They produce sheaths, and are
motile by way of flagella. Cells are straight rods, Gram-negative and motile
by flagella. Growth is filamentous under natural conditions, owing to the formation
of tubular sheaths that surround single and linear chains of cells. Sheaths
are produced by excretion of fibrillar polymeric substances that are crosslinked
to form a mesh-like fabric closely fitting to the cells. In contrast to slimes
or capsules, this matrix is not in intimate contact with the cells.A linear
arrangement of single cells within a tubular sheath enables bacteria to form
filaments, without actual enlargement of cell size. It has been shown that filamentous
growth is an effective strategy of bacteria to exceed the size limit of particles
edible by protozoa, thereby allowing them to escape from grazing (Sommaruga
and Psenner, 1995). In addition, sheaths provide cells with physical protection
from infection by bacteriophages (Winston and Thompson, 1979), bacterial predators
(Venosa, 1975), and bacterivorous metazoa. Obviously tough sheaths impregnated
with ferric oxides cannot be consumed by metazoa and are not penetrated by bdellovibrios
or phages.At circumneutral pH, biological oxidation of iron is difficult to
distinguish from the chemical oxidation by oxygen. Only with the identification
of an iron-oxidizing protein with a molecular weight of 150 kDa in spent culture
medium of the sheathless strain Leptothrix discophora SS-1, the capability of
biological iron-oxidation in Leptothrix species could be clearly demonstrated
(Corstjens et al., 1992).
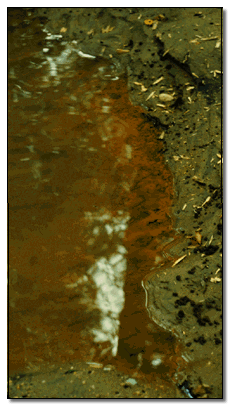
Websites relevant to Fe oxidation:
http://www.splammo.net/bact102/102ironbact.html
http://pubs.usgs.gov/publications/text/Norriemicrobes.html
http://www.riversalive.org/iron_bacteria.htm
Thiobacillus
can use ferrous iron from the oxidation of reduced sulfur compounds if provided
with a terminal electron acceptor, but the conditions required (e.g., low pH)
may vary somewhat.
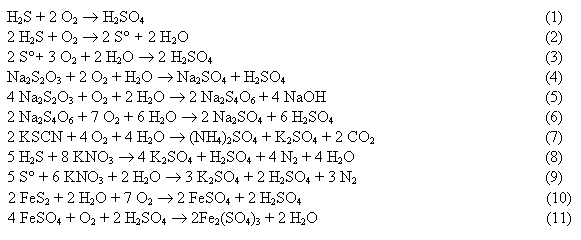
Certain
strains of purple bacteria use ferrous iron as the sole electron donor for photosynthesis.
Two different types of purple bacteria, represented by strains L7 and SW2, were
isolated which oxidized colorless ferrous iron under anoxic conditions in the
light to brown ferric iron. Strain L7 had rod-shaped, nonmotile cells (1.3 by
2 to 3 microns) which frequently formed gas vesicles. In addition to ferrous
iron, strain L7 used H2 + CO2, acetate, pyruvate, and glucose as substrate for
phototrophic growth. Strain SW2 had small rod-shaped, nonmotile cells (0.5 by
1 to 1.5 microns). Besides ferrous iron, strain SW2 utilized H2 + CO2, monocarboxylic
acids, glucose, and fructose. Neither strain utilized free sulfide; however,
both strains grew on black ferrous sulfide (FeS) which was converted to ferric
iron and sulfate. Strains L7 and SW2 grown photoheterotrophically without ferrous
iron were purple to brownish red and yellowish brown, respectively; absorption
spectra revealed peaks characteristic of bacteriochlorophyll a. The closest
phototrophic relatives of strains L7 and SW2 so far examined on the basis of
16S rRNA sequences were species of the genera Chromatium (gamma subclass of
proteobacteria) and Rhodobacter (alpha subclass), respectively. In mineral medium,
the new isolates formed 7.6 g of cell dry mass per mol of Fe(II) oxidized, which
is in good agreement with a photoautotrophic utilization of ferrous iron as
electron donor for CO2 fixation. Dependence of ferrous iron oxidation on light
and CO2 was also demonstrated in dense cell suspensions. In media containing
both ferrous iron and an organic substrate (e.g., acetate, glucose), strain
L7 utilized ferrous iron and the organic compound simultaneously; in contrast,
strain SW2 started to oxidize ferrous iron only after consumption of the organic
electron donor. Ferrous iron oxidation by anoxygenic phototrophs is understandable
in terms of energetics. In contrast to the Fe3+/Fe2+ pair (E0 = +0.77 V) existing
in acidic solutions, the relevant redox pair at pH 7 in bicarbonate-containing
environments, Fe(OH)3 + HCO3-/FeCO3, has an E0' of +0.2 V. Ferrous iron at pH
7 can therefore donate electrons to the photosystem of anoxygenic phototrophs,
which in purple bacteria has a midpoint potential around +0.45 V. The existence
of ferrous iron-oxidizing anoxygenic phototrophs may offer an explanation for
the deposition of early banded-iron formations in an assumed anoxic biosphere
in Archean times